Utilisateur:Oimabe/Cooling tower
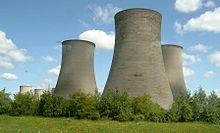


Une tour de refroidissement est un dispositif de rejet de chaleur qui rejette la chaleur résiduelle dans l'atmosphère par le refroidissement d'un flux d'eau à une température inférieure. Les tours de refroidissement peuvent utiliser l’évaporation de l’eau pour évacuer la chaleur du procédé et refroidir le fluide de travail à proximité de la température du thermomètre mouillé ou, dans le cas des tours de refroidissement à circuit fermé, uniquement température de l'air sec.
Les applications courantes incluent le refroidissement de l'eau de circulation utilisée dans les raffineries de pétrole, les usines pétrochimiques et autres usines chimiques, les centrales thermiques et les systèmes CVC pour le refroidissement des bâtiments. La classification est basée sur le type d'induction d'air dans la tour: les principaux types de tours de refroidissement sont les tours de refroidissement à tirage naturel et à tirage induit.
La taille des tours de refroidissement varie de petites unités de toit à de très grandes structures hyperboloïdes (comme sur l'image adjacente) pouvant atteindre 200 mètres (660 pieds) de hauteur et 100 mètres (330 pieds) de diamètre ou des structures rectangulaires pouvant avoir plus de 40 mètres de haut et 80 mètres de long. Les tours de refroidissement hyperboloïdes sont souvent associées aux centrales nucléaires,[1] bien qu'ils soient également utilisés dans certaines centrales au charbon et, dans une certaine mesure, dans certaines grandes usines chimiques et industrielles. Bien que ces grandes tours soient très en vue, la grande majorité des tours de refroidissement sont beaucoup plus petites, y compris de nombreuses unités installées sur ou près des bâtiments pour évacuer la chaleur de la climatisation.
Histoire modifier

Les tours de refroidissement ont vu le jour au 19ème siècle à travers le développement de condenseurs destinés à être utilisés avec la machine à vapeur.[2] Les condenseurs utilisent de l'eau relativement froide, par divers moyens, pour condenser la vapeur sortant des cylindres ou des turbines. Cela réduit la contre-pression, ce qui réduit la consommation de vapeur et donc la consommation de carburant, tout en augmentant la puissance et en recyclant l'eau des chaudières..[3] Cependant, les condenseurs nécessitent une quantité suffisante d'eau de refroidissement, sans laquelle ils ne sont pas pratiques..[4][5] On estime que la consommation d'eau de refroidissement par les usines de traitement et les centrales électriques à l'intérieur des terres réduira la disponibilité de l'énergie pour la majorité des centrales thermiques d'ici 2040-2069..[6] Bien que l’utilisation de l’eau ne soit pas un problème avec les moteurs maritimes, elle constitue une limitation importante pour de nombreux systèmes terrestres./
Au tournant du XXe siècle, plusieurs méthodes de recyclage de l'eau de refroidissement par évaporation étaient utilisées dans les zones dépourvues d'approvisionnement en eau, ainsi que dans les zones urbaines où les réseaux de distribution d'eau municipaux n'étaient pas suffisants. fiable en période de demande; ou autrement suffisant pour répondre aux besoins de refroidissement.[2][5] Dans les zones avec des terres disponibles, les systèmes ont pris la forme de bassins de refroidissement; dans les zones avec des terres limitées, comme dans les villes, ils ont pris la forme de tours de refroidissement.[4][7]./
Ces premières tours ont été positionnées soit sur les toits des bâtiments, soit en tant que structures autonomes, alimentées en air par les ventilateurs ou en fonction du flux d'air naturel[4][7]. Un manuel de génie américain datant de 1911 décrivait un dessin comme «une coquille circulaire ou rectangulaire de plaque de lumière - en fait, une cheminée beaucoup raccourcie verticalement (de 20 à 40 pieds de haut) et très agrandie latéralement. on distribue des auges auxquelles on doit pomper l'eau du condenseur, d'où elles coulent par-dessus des "nattes" faites de lattes de bois ou de tamis tissés qui remplissent l'espace à l'intérieur de la tour. "[7].
Une tour de refroidissement hyperbolique a été brevetée en 1918 par les ingénieurs néerlandais Frederik van Iterson et Gerard Kuypers[8]. Les premières tours de refroidissement hyperboloïdes ont été construites en 1918 près de Heerlen. Les premiers au Royaume-Uni ont été construits en 1924 à la centrale électrique de Lister Drive à Liverpool, en Angleterre, pour refroidir l'eau utilisée dans une centrale électrique alimentée au charbon[9].
Classification par usage/ modifier
Heating, ventilation and air conditioning (HVAC) modifier

Une tour de refroidissement HVAC (chauffage, ventilation et climatisation) est utilisée pour éliminer («rejeter») la chaleur indésirable d’un refroidisseur. Les refroidisseurs à condensation par eau consomment généralement moins d’énergie que les refroidisseurs à condensation par air en raison du rejet de chaleur dans l’eau de la tour à la température du bulbe humide ou à proximité de celle-ci. Les refroidisseurs à condensation par air doivent rejeter la chaleur à la température plus élevée du thermomètre sec et avoir ainsi une efficacité moyenne inférieure du cycle d'inversion de Carnot. Dans les régions à climat chaud, les grands immeubles de bureaux, les hôpitaux et les écoles utilisent généralement une ou plusieurs tours de refroidissement dans leurs systèmes de climatisation. En règle générale, les tours de refroidissement industrielles sont beaucoup plus grandes que les tours de CVC./
L'utilisation de CVC d'une tour de refroidissement associe la tour de refroidissement à un refroidisseur à condensation par eau ou à un condenseur à eau. Une tonne de climatisation est définie comme la suppression de 12 000 BTU/heure (3500 W). La tonne équivalente du côté de la tour de refroidissement rejette en réalité environ 15 000 BTU / heure (4400 W) en raison de la chaleur résiduelle supplémentaire équivalente à l'énergie nécessaire pour entraîner le compresseur du refroidisseur. Cette tonne équivalente est définie comme le rejet de chaleur dans le refroidissement de 3 gallons US / minute (1500 livres / heure) d'eau 10 ° F (6 ° C), soit 15 000 BTU / heure, en supposant un coefficient de performance du refroidisseur (COP). de 4.0.[10] Ce COP est équivalent à un ratio d'efficacité énergétique (EER) de 14.
Les tours de refroidissement sont également utilisées dans les systèmes de CVC dotés de plusieurs pompes à chaleur à source d'eau partageant une boucle d'eau commune. Dans ce type de système, l'eau qui circule dans la boucle d'eau élimine la chaleur du condenseur des pompes à chaleur lorsque les pompes à chaleur fonctionnent en mode de refroidissement, puis la tour de refroidissement externe est utilisée pour éliminer la chaleur de la boucle d'eau et rejeter à l'atmosphère. En revanche, lorsque les pompes à chaleur fonctionnent en mode chauffage, les condenseurs extraient la chaleur de l'eau de la boucle et la rejettent dans l'espace à chauffer. Lorsque la boucle d'eau est principalement utilisée pour fournir de la chaleur au bâtiment, la tour de refroidissement est normalement fermée (et peut être vidangée ou hivernisée pour éviter les dommages dus au gel) et la chaleur est fournie par d'autres moyens, généralement des chaudières séparées..
Tours de refroidissement industrielles modifier

Les tours de refroidissement industrielles peuvent être utilisées pour extraire la chaleur de diverses sources telles que des machines ou des matériaux de processus chauffés. Les grandes tours de refroidissement industrielles sont principalement utilisées pour éliminer la chaleur absorbée par les systèmes d’eau de refroidissement en circulation utilisés dans les centrales électriques, les raffineries de pétrole, les usines pétrochimiques, les usines de traitement du gaz naturel, les usines de transformation des aliments, les semi-conducteurs et d’autres installations industrielles. des installations telles que des condenseurs de colonnes de distillation, pour refroidir un liquide dans une cristallisation, etc..[11] Le débit de circulation de l'eau de refroidissement dans une centrale au charbon typique de 700 MW avec une tour de refroidissement s'élève à environ 71 600 mètres cubes par heure (315 000 gallons américains par minute).[12] et la circulation de l’eau nécessite un taux de réalimentation en eau d’alimentation d’environ 5% (soit 3 600 mètres cubes par heure) ./
Si cette même usine ne possédait pas de tour de refroidissement et utilisait de l'eau de refroidissement à passage unique, elle aurait besoin d'environ 100 000 mètres cubes par jour[13]. Une grande consommation d'eau de refroidissement tue généralement des millions de poissons et de larves chaque année, car les organismes sont touchés par les filtres d'aspiration[14]. Une grande quantité d’eau devrait être renvoyée en permanence dans l’océan, le lac ou la rivière d’où elle a été obtenue et continuellement ravitaillée à l’usine. En outre, le rejet de grandes quantités d'eau chaude peut augmenter la température du fleuve ou du lac récepteur à un niveau inacceptable pour l'écosystème local. Les températures élevées de l'eau peuvent tuer les poissons et d'autres organismes aquatiques (voir pollution thermique), ou peuvent également entraîner une augmentation des organismes indésirables tels que les espèces envahissantes de moules zébrées ou d'algues. Une tour de refroidissement sert à dissiper la chaleur dans l'atmosphère et la diffusion du vent et de l'air diffuse la chaleur sur une surface beaucoup plus grande que l'eau chaude ne peut distribuer la chaleur dans une masse d'eau. L'eau de refroidissement par évaporation ne peut pas être utilisée à des fins ultérieures (autre que la pluie quelque part), tandis que l'eau de refroidissement de surface uniquement peut être réutilisée. Certaines centrales au charbon et centrales nucléaires situées dans les zones côtières utilisent l’eau de mer à passage unique. Mais même là, la sortie d’eau de décharge en mer nécessite une conception très soigneuse pour éviter les problèmes d’environnement./
Les raffineries de pétrole ont également de très grands systèmes de tours de refroidissement. Une grande raffinerie typique traitant 40 000 tonnes métriques de pétrole brut par jour (300 000 barils (48 000 m3) par jour) fait circuler environ 80 000 mètres cubes d'eau par heure dans son système de tour de refroidissement.
La tour de refroidissement la plus haute du monde est la tour de refroidissement de 202 mètres (662,7296598 pi) de la centrale thermique de Kalisindh à Jhalawar, au Rajasthan, en Inde[15].
Classement par construction. modifier
Type préassemblé modifier


Ces types de tours de refroidissement sont préassemblés en usine et peuvent être simplement transportés sur des camions, car ils sont des machines compactes. La capacité des tours de type colis est limitée et, pour cette raison, elles sont généralement préférées par les installations à faible rejet thermique, telles que les usines de transformation des aliments, les usines textiles, certaines usines de traitement chimique, les hôpitaux, les hôtels, les centres commerciaux etc/.
En raison de leur utilisation fréquente dans ou près des zones résidentielles, le contrôle du niveau sonore est un problème relativement plus important pour les tours de refroidissement de type paquet/.
Type de montage sur le terrain modifier
Les installations telles que les centrales électriques, les usines de traitement de l'acier, les raffineries de pétrole ou les usines pétrochimiques installent généralement des tours de refroidissement de type érigées sur le terrain en raison de leur plus grande capacité de rejet de chaleur. Les tours érigées sur le terrain sont généralement beaucoup plus grandes que les tours de refroidissement de type paquet/.
Une tour de refroidissement type érigée sur le terrain a une structure en plastique renforcé de fibres pultrudées (FRP), un revêtement en FRP, une unité mécanique pour le tirage d'air, un éliminateur de gouttes et un remplissage/.
Méthodes de transfert de chaleur; modifier
En ce qui concerne le mécanisme de transfert de chaleur utilisé, les principaux types sont les suivants:
- Les tours de refroidissement sèches fonctionnent par transfert de chaleur à travers une surface qui sépare le fluide de travail de l'air ambiant, par exemple dans un échangeur de chaleur à tube en utilisant un transfert de chaleur par convection. Ils n'utilisent pas l'évaporation..
- Les tours de refroidissement par voie humide (ou les tours de refroidissement à circuit ouvert) fonctionnent selon le principe du refroidissement par évaporation. Le fluide de travail et le fluide évaporé (généralement de l’eau) sont identiques..
- Les refroidisseurs de fluide (ou tours de refroidissement à circuit fermé) sont des hybrides qui font passer le fluide de travail à travers un faisceau de tubes, sur lequel de l'eau propre est pulvérisée et un tirage induit par un ventilateur. Les performances de transfert de chaleur qui en résultent sont bien plus proches de celles d’une tour de refroidissement par voie humide, avec l’avantage procuré par un refroidisseur à sec de protéger le fluide de travail de l’exposition et de la contamination de l’environnement..
Dans une tour de refroidissement humide (ou une tour de refroidissement à circuit ouvert), l'eau chaude peut être refroidie à une température inférieure à la température de bulbe sec de l'air ambiant, si l'air est relativement sec (voir point de rosée et psychrométrie). Lorsque l'air ambiant passe dans un flux d'eau, une petite partie de celle-ci s'évapore. L'énergie nécessaire à l'évaporation de cette partie de l'eau provient de la masse restante d'eau, ce qui réduit sa température. Environ 970 BTU d'énergie thermique sont absorbés pour chaque livre d'eau évaporée (2 MJ / kg). L'évaporation entraîne une saturation de l'air, abaissant la température de l'eau traitée par la tour à une valeur proche de la température de bulbe humide, inférieure à la température ambiante de bulbe sec, différence déterminée par l'humidité initiale de l'air ambiant..
Pour obtenir de meilleures performances (plus de refroidissement), un milieu appelé remplissage est utilisé pour augmenter la surface et le temps de contact entre les flux d'air et d'eau. Le remplissage par éclaboussures est constitué de matériaux placés pour interrompre le flux d’eau et provoquer des éclaboussures. Le remplissage de film est composé de fines feuilles de matériau (généralement du PVC) sur lesquelles l'eau s'écoule. Les deux méthodes créent une surface et un temps de contact accrus entre le fluide (eau) et le gaz (air), afin d'améliorer le transfert de chaleur..
Méthodes de génération de flux d'air modifier

With respect to drawing air through the tower, there are three types of cooling towers:
- Tirage naturel - Utilise la flottabilité via une grande cheminée. L'air chaud et humide augmente naturellement en raison de la différence de densité par rapport à l'air extérieur sec et plus froid. L'air chaud et humide est moins dense que l'air plus sec à la même pression. Cette flottabilité de l'air humide produit un courant d'air ascendant à travers la tour./
- Tirage mécanique - Utilise des moteurs de ventilateur à moteur pour forcer ou aspirer de l'air à travers la tour.
- Tirage induit - Une tour à tirage mécanique avec un ventilateur à la sortie (au sommet) qui aspire l'air à travers la tour. Le ventilateur fait sortir l'air chaud et humide de la décharge. Cela produit de faibles vitesses d’entrée et de sortie d’air, ce qui réduit le risque de recirculation dans lequel l’air rejeté retourne dans la prise d’air. Cet arrangement ventilateur / ailette est également connu sous le nom de tirage.
- Tirage forcé - Tour à tirage mécanique avec un ventilateur de type soufflante à l'admission. Le ventilateur force l'air dans la tour, créant des vitesses d'air élevées à l'entrée et à la sortie. La faible vitesse de sortie est beaucoup plus susceptible de recirculation. Avec le ventilateur sur l'admission d'air, le ventilateur est plus susceptible aux complications dues au gel. Un autre inconvénient est qu'une conception à tirage forcé nécessite généralement plus de puissance motrice qu'une conception à tirage induit équivalente. L'avantage de la conception à tirage forcé est sa capacité à travailler avec une pression statique élevée. De telles installations peuvent être installées dans des espaces plus confinés et même dans certaines situations d'intérieur. Cette géométrie éventail / ailette est également appelée soufflage..
- Ventilation naturelle assistée par ventilateur - Un type hybride qui ressemble à une configuration à tirage naturel, bien que la ventilation soit assistée par un ventilateur.
Les tours de refroidissement hyperboloïdiques (parfois appelées à tort hyperboliques) sont devenues la norme de conception pour toutes les tours de refroidissement à tirage naturel en raison de leur résistance structurelle et de l'utilisation minimale de matériau. La forme hyperboloïde aide également à accélérer le flux d’air convectif vers le haut, améliorant ainsi l’efficacité du refroidissement. Ces conceptions sont généralement associées aux centrales nucléaires. Cependant, cette association est trompeuse, car le même type de tours de refroidissement est souvent utilisé dans les grandes centrales au charbon. À l'inverse, toutes les centrales nucléaires ne disposent pas de tours de refroidissement et certaines refroidissent leurs échangeurs de chaleur avec de l'eau de lac, de rivière ou d'océan.
- CleanEnergy Footprints (cleanenergy.org). Identifying Nuclear Reactors in Google Earth Retrieved 5/19/2014
- International Correspondence Schools, A Textbook on Steam Engineering, Scranton, Pa., International Textbook Co., (lire en ligne)
- Steam-Engine Principles and Practice, New York, McGraw-Hill, , 283–286 p. (lire en ligne)
- Robert Culbertson Hays Heck, The Steam Engine and Turbine: A Text-Book for Engineering Colleges, New York, D. Van Nostrand, , 569–570 p. (lire en ligne)
- Egbert P. Watson, « Power plant and allied industries », The Engineer (with Which is Incorporated Steam Engineering), Chicago, Taylor Publishing Co., vol. 43, no 1, , p. 69–72 (lire en ligne)
- Michelle T. H. van Vliet et David Wiberg, « Power-generation system vulnerability and adaptation to changes in climate and water resources », (DOI 10.1038/nclimate2903, consulté le )
- Walter B. Snow, The Steam Engine: A Practical Guide to the Construction, Operation, and care of Steam Engines, Steam Turbines, and Their Accessories, Chicago, American School of Correspondence, , 43–46 p. (lire en ligne)
- UK Patent No. 108,863
- "Power Plant Cooling Towers Like Big Milk Bottle" Popular Mechanics, February 1930 bottom-left of pg 201
- Nicholas Cheremisinoff, Handbook of Chemical Processing Equipment, Butterworth-Heinemann, (ISBN 9780080523828), p. 69
- {{Cite report}} : paramètre
titre
manquant Document No. EPA/310-R-97-007. p. 79. - Cooling System Retrofit Costs EPA Workshop on Cooling Water Intake Technologies, John Maulbetsch, Maulbetsch Consulting, May 2003
- Thomas J. Feeley, III, Lindsay Green, James T. Murphy, Jeffrey Hoffmann, and Barbara A. Carney (2005). "Department of Energy/Office of Fossil Energy’s Power Plant Water Management R&D Program." « https://web.archive.org/web/20070927104349/http://204.154.137.14/technologies/coalpower/ewr/pubs/IEP_Power_Plant_Water_R%26D_Final_1.pdf »(Archive.org • Wikiwix • Archive.is • Google • Que faire ?), Wayback Machine« https://web.archive.org/web/20070927104349/http://204.154.137.14/technologies/coalpower/ewr/pubs/IEP_Power_Plant_Water_R%26D_Final_1.pdf »(Archive.org • Wikiwix • Archive.is • Google • Que faire ?), U.S. Department of Energy, July 2005.
- The Indian Point Energy Center cooling system kills over a billion fish eggs and larvae annually. (en) Patrick McGeehan, « Fire Prompts Renewed Calls to Close the Indian Point Nuclear Plant », New York Times, (lire en ligne)
- Comansa Jie builds the world’s highest cooling towers
Des rendements thermiques allant jusqu'à 92% ont été observés dans les tours de refroidissement hybrides.[1]
Catégorisation par flux air-eau modifier
Crossflow modifier



Généralement, coûts initiaux et à long terme moins élevés, principalement en raison des besoins en pompes./
Le flux transversal est une conception dans laquelle le flux d'air est dirigé perpendiculairement au flux d'eau (voir le schéma à gauche). Le flux d'air pénètre dans une ou plusieurs faces verticales de la tour de refroidissement pour rencontrer le matériau de remplissage. L'eau s'écoule (perpendiculairement à l'air) à travers le remblai par gravité. L'air continue à travers le remblai et passe ainsi le flux d'eau dans un plénum ouvert. Enfin, un ventilateur force l'air dans l'atmosphère./
A distribution or hot water basin consisting of a deep pan with holes or nozzles in its bottom is located near the top of a crossflow tower. Gravity distributes the water through the nozzles uniformly across the fill material.
Advantages of the crossflow design:
- Gravity water distribution allows smaller pumps and maintenance while in use.
- Non-pressurized spray simplifies variable flow.
Disadvantages of the crossflow design:
- More prone to freezing than counterflow designs.
- Variable flow is useless in some conditions.
- More prone to dirt buildup in the fill than counterflow designs, especially in dusty or sandy areas.
Counterflow modifier

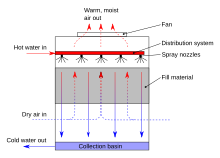

In a counterflow design, the air flow is directly opposite to the water flow (see diagram at left). Air flow first enters an open area beneath the fill media, and is then drawn up vertically. The water is sprayed through pressurized nozzles near the top of the tower, and then flows downward through the fill, opposite to the air flow.
Advantages of the counterflow design:
- Spray water distribution makes the tower more freeze-resistant.
- Breakup of water in spray makes heat transfer more efficient.
Disadvantages of the counterflow design:
- Typically higher initial and long-term cost, primarily due to pump requirements.
- Difficult to use variable water flow, as spray characteristics may be negatively affected.
- Typically noisier, due to the greater water fall height from the bottom of the fill into the cold water basin
Common aspects modifier
Common aspects of both designs:
- The interactions of the air and water flow allow a partial equalization of temperature, and evaporation of water.
- The air, now saturated with water vapor, is discharged from the top of the cooling tower.
- A "collection basin" or "cold water basin" is used to collect and contain the cooled water after its interaction with the air flow.
Both crossflow and counterflow designs can be used in natural draft and in mechanical draft cooling towers.
Wet cooling tower material balance modifier
Quantitatively, the material balance around a wet, evaporative cooling tower system is governed by the operational variables of make-up volumetric flow rate, evaporation and windage losses, draw-off rate, and the concentration cycles.[2][3]
In the adjacent diagram, water pumped from the tower basin is the cooling water routed through the process coolers and condensers in an industrial facility. The cool water absorbs heat from the hot process streams which need to be cooled or condensed, and the absorbed heat warms the circulating water (C). The warm water returns to the top of the cooling tower and trickles downward over the fill material inside the tower. As it trickles down, it contacts ambient air rising up through the tower either by natural draft or by forced draft using large fans in the tower. That contact causes a small amount of the water to be lost as windage/drift (W) and some of the water (E) to evaporate. The heat required to evaporate the water is derived from the water itself, which cools the water back to the original basin water temperature and the water is then ready to recirculate. The evaporated water leaves its dissolved salts behind in the bulk of the water which has not been evaporated, thus raising the salt concentration in the circulating cooling water. To prevent the salt concentration of the water from becoming too high, a portion of the water is drawn off/blown down (D) for disposal. Fresh water make-up (M) is supplied to the tower basin to compensate for the loss of evaporated water, the windage loss water and the draw-off water.

Using these flow rates and concentration dimensional units:
M | = Make-up water in m3/h |
C | = Circulating water in m3/h |
D | = Draw-off water in m3/h |
E | = Evaporated water in m3/h |
W | = Windage loss of water in m3/h |
X | = Concentration in ppmw (of any completely soluble salts ... usually chlorides) |
XM | = Concentration of chlorides in make-up water (M), in ppmw |
XC | = Concentration of chlorides in circulating water (C), in ppmw |
Cycles | = Cycles of concentration = XC / XM (dimensionless) |
ppmw | = parts per million by weight |
A water balance around the entire system is then:[3]
Since the evaporated water (E) has no salts, a chloride balance around the system is:[3]
and, therefore:[3]
From a simplified heat balance around the cooling tower:
where: | |
HV | = latent heat of vaporization of water = 2260 kJ / kg |
ΔT | = water temperature difference from tower top to tower bottom, in °C |
cp | = specific heat of water = 4.184 kJ / (kg°C) |
Windage (or drift) losses (W) is the amount of total tower water flow that is entrained in the flow of air to the atmosphere. From large-scale industrial cooling towers, in the absence of manufacturer's data, it may be assumed to be:
- W = 0.3 to 1.0 percent of C for a natural draft cooling tower without windage drift eliminators
- W = 0.1 to 0.3 percent of C for an induced draft cooling tower without windage drift eliminators
- W = about 0.005 percent of C (or less) if the cooling tower has windage drift eliminators
- W = about 0.0005 percent of C (or less) if the cooling tower has windage drift eliminators and uses sea water as make-up water.
Cycles of concentration modifier
Cycle of concentration represents the accumulation of dissolved minerals in the recirculating cooling water. Discharge of draw-off (or blowdown) is used principally to control the buildup of these minerals.
The chemistry of the make-up water, including the amount of dissolved minerals, can vary widely. Make-up waters low in dissolved minerals such as those from surface water supplies (lakes, rivers etc.) tend to be aggressive to metals (corrosive). Make-up waters from ground water supplies (such as wells) are usually higher in minerals, and tend to be scaling (deposit minerals). Increasing the amount of minerals present in the water by cycling can make water less aggressive to piping; however, excessive levels of minerals can cause scaling problems.

As the cycles of concentration increase, the water may not be able to hold the minerals in solution. When the solubility of these minerals have been exceeded they can precipitate out as mineral solids and cause fouling and heat exchange problems in the cooling tower or the heat exchangers. The temperatures of the recirculating water, piping and heat exchange surfaces determine if and where minerals will precipitate from the recirculating water. Often a professional water treatment consultant will evaluate the make-up water and the operating conditions of the cooling tower and recommend an appropriate range for the cycles of concentration. The use of water treatment chemicals, pretreatment such as water softening, pH adjustment, and other techniques can affect the acceptable range of cycles of concentration.
Concentration cycles in the majority of cooling towers usually range from 3 to 7. In the United States, many water supplies use well water which has significant levels of dissolved solids. On the other hand, one of the largest water supplies, for New York City, has a surface rainwater source quite low in minerals; thus cooling towers in that city are often allowed to concentrate to 7 or more cycles of concentration.
Since higher cycles of concentration represent less make-up water, water conservation efforts may focus on increasing cycles of concentration.[4] Highly treated recycled water may be an effective means of reducing cooling tower consumption of potable water, in regions where potable water is scarce.[5]
Maintenance modifier
Les surfaces présentant un biofilm visible (c'est-à-dire de la boue) doivent être nettoyées. Les niveaux de désinfectant et d’autres produits chimiques dans les tours de refroidissement et les spas doivent être entretenus et surveillés en permanenceref>Beychok, Milton R., Developing a Water Management Program to Reduce Legionella Growth & Spread in Buildings: A Practical Guide to Implementing Industry Standards, CDC, (lire en ligne), p. 13 {17 of 32}</ref>.
Des contrôles réguliers de la qualité de l'eau (en particulier des niveaux de bactéries aérobies) à l'aide de dipslides doivent être pratiqués car la présence d'autres organismes peut favoriser la légionellose en produisant les nutriments organiques dont elle a besoin pour se développer[6].
Water treatment modifier
Outre le traitement de l'eau de refroidissement en circulation dans les grands systèmes de tour de refroidissement industriels afin de minimiser l'entartrage et l'encrassement, l'eau doit également être filtrée pour éliminer les particules et être dosée avec du biocides et Algicides pour prévenir les excroissances susceptibles d’interférer avec le flux continu de l’eau[2]. Dans certaines conditions, un biofilm de micro-organismes tels que des bactéries, des champignons et des algues peut se développer très rapidement dans l'eau de refroidissement et peut réduire l'efficacité du transfert de chaleur de la tour de refroidissement. Le biofilm peut être réduit ou prévenu en utilisant chlore ou d'autres produits chimiques. Une pratique industrielle normale consiste à utiliser deux biocides, tels que les types oxydant et non oxydant, pour compléter leurs forces et leurs faiblesses et pour assurer un spectre d'attaque plus large. Dans la plupart des cas, on utilise un biocide oxydant continu à faible concentration, alternant ensuite avec une dose de choc périodique de biocides non oxydants[7].
Légionellose modifier

Une autre raison très importante d'utiliser des biocides dans les tours de refroidissement est d'empêcher la croissance de Legionella , y compris d'espèces causant la légionellose ou la maladie du légionnaire, notamment L. pneumophila ,[8] ou Mycobacterium avium .[9] The various Legionella species are the cause of Legionnaires' disease in humans and transmission is via exposure to aerosols—the inhalation of mist droplets containing the bacteria. Common sources of Legionella include cooling towers used in open recirculating evaporative cooling water systems, domestic hot water systems, fountains, and similar disseminators that tap into a public water supply. Natural sources include freshwater ponds and creeks.[10][11]
French researchers found that Legionella bacteria travelled up to 6 kilomètres (3,728227152 mi) through the air from a large contaminated cooling tower at a petrochemical plant in Pas-de-Calais, France. That outbreak killed 21 of the 86 people who had a laboratory-confirmed infection.[12]
Drift (or windage) is the term for water droplets of the process flow allowed to escape in the cooling tower discharge. Drift eliminators are used in order to hold drift rates typically to 0.001–0.005% of the circulating flow rate. A typical drift eliminator provides multiple directional changes of airflow to prevent the escape of water droplets. A well-designed and well-fitted drift eliminator can greatly reduce water loss and potential for Legionella or water treatment chemical exposure.
The CDC does not recommend that health-care facilities regularly test for the Legionella pneumophila bacteria. Scheduled microbiologic monitoring for Legionella remains controversial because its presence is not necessarily evidence of a potential for causing disease. The CDC recommends aggressive disinfection measures for cleaning and maintaining devices known to transmit Legionella, but does not recommend regularly-scheduled microbiologic assays for the bacteria. However, scheduled monitoring of potable water within a hospital might be considered in certain settings where persons are highly susceptible to illness and mortality from Legionella infection (e.g. hematopoietic stem cell transplantation units, or solid organ transplant units). Also, after an outbreak of legionellosis, health officials agree that monitoring is necessary to identify the source and to evaluate the efficacy of biocides or other prevention measures.[13]
Studies have found Legionella in 40% to 60% of cooling towers.[14]
Many governmental agencies, cooling tower manufacturers and industrial trade organizations have developed design and maintenance guidelines for preventing or controlling the growth of Legionella in cooling towers. Below is a list of sources for such guidelines:
- « Centers for Disease Control and Prevention (CDC) » (4.99 MB) – Procedure for Cleaning Cooling Towers and Related Equipment (pages 225 and 226)
- « Cooling Technology Institute » (240 KB) – Best Practices for Control of Legionella, July, 2006
- « Association of Water Technologies » [archive du ]« Association of Water Technologies » [archive du ] (964 KB) – Legionella 2003: An Update and Statement
- « California Energy Commission » (194 KB) – Cooling Water Management Program Guidelines For Wet and Hybrid Cooling Towers at Power Plants
- « SPX Cooling Technologies » (119 KB) – Cooling Towers Maintenance Procedures
- « SPX Cooling Technologies » (789 KB) – ASHRAE Guideline 12-2000 – Minimizing the Risk of Legionellosis
- « SPX Cooling Technologies » (83.1 KB) – Cooling Tower Inspection Tips {especially page 3 of 7}
- « Tower Tech Modular Cooling Towers » (109 KB) – Legionella Control
- « GE Infrastructure Water & Process Technologies Betz Dearborn » [archive du ]« GE Infrastructure Water & Process Technologies Betz Dearborn » [archive du ] (195 KB) – Chemical Water Treatment Recommendations For Reduction of Risks Associated with Legionella in Open Recirculating Cooling Water Systems
- Blow-out — Water droplets blown out of the cooling tower by wind, generally at the air inlet openings. Water may also be lost, in the absence of wind, through splashing or misting. Devices such as wind screens, louvers, splash deflectors and water diverters are used to limit these losses.

Terminology modifier

- Windage or Drift — Water droplets that are carried out of the cooling tower with the exhaust air. Drift droplets have the same concentration of impurities as the water entering the tower. The drift rate is typically reduced by employing baffle-like devices, called drift eliminators, through which the air must travel after leaving the fill and spray zones of the tower. Drift can also be reduced by using warmer entering cooling tower temperatures.
- Blow-out — Water droplets blown out of the cooling tower by wind, generally at the air inlet openings. Water may also be lost, in the absence of wind, through splashing or misting. Devices such as wind screens, louvers, splash deflectors and water diverters are used to limit these losses.
- Windage or Drift — Water droplets that are carried out of the cooling tower with the exhaust air. Drift droplets have the same concentration of impurities as the water entering the tower. The drift rate is typically reduced by employing baffle-like devices, called drift eliminators, through which the air must travel after leaving the fill and spray zones of the tower. Drift can also be reduced by using warmer entering cooling tower temperatures.
- Plume — The stream of saturated exhaust air leaving the cooling tower. The plume is visible when water vapor it contains condenses in contact with cooler ambient air, like the saturated air in one's breath fogs on a cold day. Under certain conditions, a cooling tower plume may present fogging or icing hazards to its surroundings. Note that the water evaporated in the cooling process is "pure" water, in contrast to the very small percentage of drift droplets or water blown out of the air inlets.
- Draw-off or Blow-down — The portion of the circulating water flow that is removed (usually discharged to a drain) in order to maintain the amount of Total Dissolved Solids (TDS) and other impurities at an acceptably low level. Higher TDS concentration in solution may result from greater cooling tower efficiency. However the higher the TDS concentration, the greater the risk of scale, biological growth and corrosion. The amount of blow-down is primarily designated by measuring by the electrical conductivity of the circulating water. Biological growth, scaling and corrosion can be prevented by chemicals (respectively, biocide, sulfuric acid, corrosion inhibitor). On the other hand, the only practical way to decrease the electrical conductivity is by increasing the amount of blow-down discharge and subsequently increasing the amount of clean make-up water.
- Zero bleed for cooling towers, also called zero blow-down for cooling towers, is a process for significantly reducing the need for bleeding water with residual solids from the system by enabling the water to hold more solids in solution.[15][16][17]
- Make-up — The water that must be added to the circulating water system in order to compensate for water losses such as evaporation, drift loss, blow-out, blow-down, etc.
- Draw-off or Blow-down — The portion of the circulating water flow that is removed (usually discharged to a drain) in order to maintain the amount of Total Dissolved Solids (TDS) and other impurities at an acceptably low level. Higher TDS concentration in solution may result from greater cooling tower efficiency. However the higher the TDS concentration, the greater the risk of scale, biological growth and corrosion. The amount of blow-down is primarily designated by measuring by the electrical conductivity of the circulating water. Biological growth, scaling and corrosion can be prevented by chemicals (respectively, biocide, sulfuric acid, corrosion inhibitor). On the other hand, the only practical way to decrease the electrical conductivity is by increasing the amount of blow-down discharge and subsequently increasing the amount of clean make-up water.
- Zero bleed for cooling towers, also called zero blow-down for cooling towers, is a process for significantly reducing the need for bleeding water with residual solids from the system by enabling the water to hold more solids in solution.[15][16][17]
- Make-up — The water that must be added to the circulating water system in order to compensate for water losses such as evaporation, drift loss, blow-out, blow-down, etc.
- Noise — Sound energy emitted by a cooling tower and heard (recorded) at a given distance and direction. The sound is generated by the impact of falling water, by the movement of air by fans, the fan blades moving in the structure, vibration of the structure, and the motors, gearboxes or drive belts.
- Approach — The approach is the difference in temperature between the cooled-water temperature and the entering-air wet bulb temperature (twb). Since the cooling towers are based on the principles of evaporative cooling, the maximum cooling tower efficiency depends on the wet bulb temperature of the air. The wet-bulb temperature is a type of temperature measurement that reflects the physical properties of a system with a mixture of a gas and a vapor, usually air and water vapor
- Noise — Sound energy emitted by a cooling tower and heard (recorded) at a given distance and direction. The sound is generated by the impact of falling water, by the movement of air by fans, the fan blades moving in the structure, vibration of the structure, and the motors, gearboxes or drive belts.
- Approach — The approach is the difference in temperature between the cooled-water temperature and the entering-air wet bulb temperature (twb). Since the cooling towers are based on the principles of evaporative cooling, the maximum cooling tower efficiency depends on the wet bulb temperature of the air. The wet-bulb temperature is a type of temperature measurement that reflects the physical properties of a system with a mixture of a gas and a vapor, usually air and water vapor
- Full-Flow Filtration — Full-flow filtration continuously strains particulates out of the entire system flow. For example, in a 100-ton system, the flow rate would be roughly 300 gal/min. A filter would be selected to accommodate the entire 300 gal/min flow rate. In this case, the filter typically is installed after the cooling tower on the discharge side of the pump. While this is the ideal method of filtration, for higher flow systems it may be cost-prohibitive.
- Range — The range is the temperature difference between the warm water inlet and cooled water exit.
- Fill — Inside the tower, fills are added to increase contact surface as well as contact time between air and water, to provide better heat transfer. The efficiency of the tower depends on the selection and amount of fill. There are two types of fills that may be used:
- Film type fill (causes water to spread into a thin film)
- Splash type fill (breaks up falling stream of water and interrupts its vertical progress)
- Side-Stream Filtration — Side-stream filtration, although popular and effective, does not provide complete protection. With side-stream filtration, a portion of the water is filtered continuously. This method works on the principle that continuous particle removal will keep the system clean. Manufacturers typically package side-stream filters on a skid, complete with a pump and controls. For high flow systems, this method is cost-effective. Properly sizing a side-stream filtration system is critical to obtain satisfactory filter performance, but there is some debate over how to properly size the side-stream system. Many engineers size the system to continuously filter the cooling tower basin water at a rate equivalent to 10% of the total circulation flow rate. For example, if the total flow of a system is 1,200 gal/min (a 400-ton system), a 120 gal/min side-stream system is specified.
- Cycle of concentration — Maximum allowed multiplier for the amount of miscellaneous substances in circulating water compared to the amount of those substances in make-up water.
- Treated timber — A structural material for cooling towers which was largely abandoned about 10 years ago.« SPX Cooling Technologies », It is still used occasionally due to its low initial costs, in spite of its short life expectancy. The life of treated timber varies a lot, depending on the operating conditions of the tower, such as frequency of shutdowns, treatment of the circulating water, etc. Under proper working conditions, the estimated life of treated timber structural members is about 10 years.
Under certain ambient conditions, plumes of water vapor (fog) can be seen rising out of the discharge from a cooling tower, and can be mistaken as smoke from a fire. If the outdoor air is at or near saturation, and the tower adds more water to the air, saturated air with liquid water droplets can be discharged, which is seen as fog. This phenomenon typically occurs on cool, humid days, but is rare in many climates. Fog and clouds associated with cooling towers can be described as homogenitus, as with other clouds of man-made origin, such as contrails and ship tracks.[18]
- Leaching — The loss of wood preservative chemicals by the washing action of the water flowing through a wood structure cooling tower.
- Pultruded FRP — A common structural material for smaller cooling towers, fibre-reinforced plastic (FRP) is known for its high corrosion-resistance capabilities. Pultruded FRP is produced using pultrusion technology, and has become the most common structural material for small cooling towers. It offers lower costs and requires less maintenance compared to reinforced concrete, which is still in use for large structures.
- Side-Stream Filtration — Side-stream filtration, although popular and effective, does not provide complete protection. With side-stream filtration, a portion of the water is filtered continuously. This method works on the principle that continuous particle removal will keep the system clean. Manufacturers typically package side-stream filters on a skid, complete with a pump and controls. For high flow systems, this method is cost-effective. Properly sizing a side-stream filtration system is critical to obtain satisfactory filter performance, but there is some debate over how to properly size the side-stream system. Many engineers size the system to continuously filter the cooling tower basin water at a rate equivalent to 10% of the total circulation flow rate. For example, if the total flow of a system is 1,200 gal/min (a 400-ton system), a 120 gal/min side-stream system is specified.
- Cycle of concentration — Maximum allowed multiplier for the amount of miscellaneous substances in circulating water compared to the amount of those substances in make-up water.
- Treated timber — A structural material for cooling towers which was largely abandoned about 10 years ago.[Quand ?] It is still used occasionally due to its low initial costs, in spite of its short life expectancy. The life of treated timber varies a lot, depending on the operating conditions of the tower, such as frequency of shutdowns, treatment of the circulating water, etc. Under proper working conditions, the estimated life of treated timber structural members is about 10 years.
- Leaching — The loss of wood preservative chemicals by the washing action of the water flowing through a wood structure cooling tower.
- Pultruded FRP — A common structural material for smaller cooling towers, fibre-reinforced plastic (FRP) is known for its high corrosion-resistance capabilities. Pultruded FRP is produced using pultrusion technology, and has become the most common structural material for small cooling towers. It offers lower costs and requires less maintenance compared to reinforced concrete, which is still in use for large structures.
Fog production modifier

Under certain ambient conditions, plumes of water vapor (fog) can be seen rising out of the discharge from a cooling tower, and can be mistaken as smoke from a fire. If the outdoor air is at or near saturation, and the tower adds more water to the air, saturated air with liquid water droplets can be discharged, which is seen as fog. This phenomenon typically occurs on cool, humid days, but is rare in many climates. Fog and clouds associated with cooling towers can be described as homogenitus, as with other clouds of man-made origin, such as contrails and ship tracks.[19]

This phenomenon can be prevented by decreasing the relative humidity of the saturated discharge air. For that purpose, in hybrid towers, saturated discharge air is mixed with heated low relative humidity air. Some air enters the tower above drift eliminator level, passing through heat exchangers. The relative humidity of the dry air is even more decreased instantly as being heated while entering the tower. The discharged mixture has a relatively lower relative humidity and the fog is invisible.
When wet cooling towers with seawater make-up are installed in various industries located in or near coastal areas, the drift of fine droplets emitted from the cooling towers contain nearly 6% sodium chloride which deposits on the nearby land areas. This deposition of sodium salts on the nearby agriculture/vegetative lands can convert them into sodic saline or sodic alkaline soils depending on the nature of the soil and enhance the sodicity of ground and surface water. The salt deposition problem from such cooling towers aggravates where national pollution control standards are not imposed or not implemented to minimize the drift emissions from wet cooling towers using seawater make-up.[20]
Respirable suspended particulate matter, of less than 10 micrometers (µm) in size, can be present in the drift from cooling towers. Larger particles above 10 µm in size are generally filtered out in the nose and throat via cilia and mucus but particulate matter smaller than 10 µm, referred to as PM10, can settle in the bronchi and lungs and cause health problems. Similarly, particles smaller than 2.5 µm, (PM2.5), tend to penetrate into the gas exchange regions of the lung, and very small particles (less than 100 nanometers) may pass through the lungs to affect other organs. Though the total particulate emissions from wet cooling towers with fresh water make-up is much less, they contain more PM10 and PM2.5 than the total emissions from wet cooling towers with sea water make-up. This is due to lesser salt content in fresh water drift (below 2,000 ppm) compared to the salt content of sea water drift (60,000 ppm).[20]

Salt emission pollution modifier
When wet cooling towers with seawater make-up are installed in various industries located in or near coastal areas, the drift of fine droplets emitted from the cooling towers contain nearly 6% sodium chloride which deposits on the nearby land areas. This deposition of sodium salts on the nearby agriculture/vegetative lands can convert them into sodic saline or sodic alkaline soils depending on the nature of the soil and enhance the sodicity of ground and surface water. The salt deposition problem from such cooling towers aggravates where national pollution control standards are not imposed or not implemented to minimize the drift emissions from wet cooling towers using seawater make-up.[20]
Respirable suspended particulate matter, of less than 10 micrometers (µm) in size, can be present in the drift from cooling towers. Larger particles above 10 µm in size are generally filtered out in the nose and throat via cilia and mucus but particulate matter smaller than 10 µm, referred to as PM10, can settle in the bronchi and lungs and cause health problems. Similarly, particles smaller than 2.5 µm, (PM2.5), tend to penetrate into the gas exchange regions of the lung, and very small particles (less than 100 nanometers) may pass through the lungs to affect other organs. Though the total particulate emissions from wet cooling towers with fresh water make-up is much less, they contain more PM10 and PM2.5 than the total emissions from wet cooling towers with sea water make-up. This is due to lesser salt content in fresh water drift (below 2,000 ppm) compared to the salt content of sea water drift (60,000 ppm).
Use as a flue-gas stack modifier

To prevent freezing, the following procedures are used:
- The use of water modulating by-pass systems is not recommended during freezing weather. In such situations, the control flexibility of variable speed motors, two-speed motors, and/or two-speed motors multi-cell towers should be considered a requirement.[21]
- Do not operate the tower unattended. Remote sensors and alarms may be installed to monitor tower conditions.
- Do not operate the tower without a heat load. Basin heaters may be used to keep the water in the tower pan at an above-freezing temperature. Heat trace ("heating tape") is a resistive heating element that is installed along water pipes to prevent freezing in cold climates.
- Maintain design water flow rate over the tower fill.
- Manipulate or reduce airflow to maintain water temperature above freezing point.[22]
Sometimes, natural draft cooling towers are constructed with structural steel in place of concrete (RCC) when the construction time of natural draft cooling tower is exceeding the construction time of the rest of the plant or the local soil is of poor strength to bear the heavy weight of RCC cooling towers or cement prices are higher at a site to opt for cheaper natural draft cooling towers made of structural steel.
Operation in freezing weather modifier
Some cooling towers (such as smaller building air conditioning systems) are shut down seasonally, drained, and winterized to prevent freeze damage.
At some modern power stations equipped with flue gas purification, such as the Großkrotzenburg Power Station and the Rostock Power Station, the cooling tower is also used as a flue-gas stack (industrial chimney), thus saving the cost of a separate chimney structure. At plants without flue gas purification, problems with corrosion may occur, due to reactions of raw flue gas with water to form acids.
Sometimes, natural draft cooling towers are constructed with structural steel in place of concrete (RCC) when the construction time of natural draft cooling tower is exceeding the construction time of the rest of the plant or the local soil is of poor strength to bear the heavy weight of RCC cooling towers or cement prices are higher at a site to opt for cheaper natural draft cooling towers made of structural steel.
Some cooling towers (such as smaller building air conditioning systems) are shut down seasonally, drained, and winterized to prevent freeze damage.
During the winter, other sites continuously operate cooling towers with
Les tours de refroidissement construit, en totalité ou en partie de matériaux combustibles peuvent le soutien interne de la propagation du feu. Ces feux peuvent devenir très intense, en raison de la grande surface-volume ratio des tours, et des feux peuvent être intensifié par convection naturelle ou assisté par ventilateur projet. Les dommages qui en résultent peuvent être suffisamment graves pour nécessiter le remplacement de l'ensemble de la cellule ou de la structure de la tour. Pour cette raison, certains codes et normes[23] recommandent que les combustibles, les tours de refroidissement être fourni avec un système automatique d'incendie système d'arrosage. Les incendies peuvent se propager à l'intérieur de la structure de la tour lorsque la cellule n'est pas en fonctionnement (comme pour l'entretien ou de la construction), et alors même que la tour est en fonctionnement, en particulier ceux de l'induit-projet de type, en raison de l'existence de la les régions relativement sèches dans les tours.[24] water leaving the tower. Basin heaters, tower draindown, and other freeze protection methods are often employed in cold climates. Operational cooling towers with malfunctions can freeze during very cold weather. Typically, freezing starts at the corners of a cooling tower with a reduced or absent heat load. Severe freezing conditions can create growing volumes of ice, resulting in increased structural loads which can cause structural damage or collapse.
Cooling towers constructed in whole or in part of combustible materials can support internal fire propagation. Such fires can become very intense, due to the high surface-volume ratio of the towers, and fires can be further intensified by natural convection or fan-assisted draft. The resulting damage can be sufficiently severe to require the replacement of the entire cell or tower structure. For this reason, some codes and standards[25] recommend that combustible cooling towers be provided with an automatic fire sprinkler system. Fires can propagate internally within the tower structure when the cell is not in operation (such as for maintenance or construction), and even while the tower is in operation, especially those of the induced-draft type, because of the existence of relatively dry areas within the towers.[26]
Being very large structures, cooling towers are susceptible to wind damage, and several spectacular failures have occurred in the past. At Ferrybridge power station on 1 November 1965, the station was the site of a major structural failure, when three of the cooling towers collapsed owing to vibrations in 85[Quand ?]85[Quand ?] winds.[réf. nécessaire] Although the structures had been built to withstand higher wind speeds, the shape of the cooling towers caused westerly winds to be funnelled into the towers themselves, creating a vortex. Three out of the original eight cooling towers were destroyed, and the remaining five were severely damaged. The towers were later rebuilt and all eight cooling towers were strengthened to tolerate adverse weather conditions. Building codes were changed to include improved structural support, and wind tunnel tests were introduced to check tower structures and configuration.« SPX Cooling Technologies: Operating Cooling Towers in Freezing Weather » [archive du ], « SPX Cooling Technologies: Operating Cooling Towers in Freezing Weather » [archive du ],
- (en) S. Gul, « Optimizing the performance of Hybrid: Induced-Forced Draft Cooling Tower », Journal of the Pakistan Institute of Chemical Engineers, vol. 43, no 2, (ISSN 1813-4092, lire en ligne)
- Beychok, Milton R., Aqueous Wastes from Petroleum and Petrochemical Plants, John Wiley and Sons, (available in many university libraries)
- Milton R. Beychok, « How To Calculate Cooling Tower Control Variables », Petroleum Processing, , p. 1452–1456
- (en) S. Gul, « Best Management Practice Cooling Tower Management », Journal of the Pakistan Institute of Chemical Engineers, Department of Energy, vol. 43, no 2, (ISSN 1813-4092, lire en ligne, consulté le )
- San Diego County Water Authority, « Technical Information for Cooling Towers Using Recycled Water », {{Article}} : paramètre «
périodique
» manquant, San Diego County Water Authority, (lire en ligne, consulté le ) - (en-US) « Cooling Water Dipslides (Box of 10) - Dip-slides.com », sur Dip-slides.com (consulté le )
- « B & V Group, Cooling Water System Chemical Range, Non-Oxidising Biocides » [archive du ] (consulté le )
- Ryan K.J.; Ray C.G. (editors), Sherris Medical Microbiology, McGraw Hill, (ISBN 0-8385-8529-9)
- Centers for Disease Control and Prevention – Emerging Infectious Diseases (page 495)
- BA Cunha, A Burillo et E Bouza, « Legionnaires' disease. », Lancet, vol. 387, no 10016, , p. 376–85 (PMID 26231463, DOI 10.1016/s0140-6736(15)60078-2)
- « Legionella (Legionnaires' Disease and Pontiac Fever) About the Disease », sur CDC, Department of Energy, (consulté le )
- Airborne Legionella May Travel Several KilometresModèle {{Lien brisé}} : paramètres «
url
» et «titre
» manquants. , (access requires free registration) - CDC Guidelines for Environmental Infection Control in Health-Care Facilities, pages 223 & 224, Water Sampling Strategies and Culture Techniques for Detecting Legionellae
- Cooling Tower Institute, July 2008. Page 5 of 12, column 1, paragraph 3. Most professional and government agencies do not recommend testing for Legionella bacteria on a routine basis.
- (194 KB)
- Institute of Industrial Engineers 1981–, Proceedings, Volume 1982, Institute of Industrial Engineers/American Institute of Industrial Engineers, (ISBN 0-8385-8529-9), p. 101
- Alton J. Mathie, A Burillo et E Bouza, « Chemical treatment for cooling water », Lancet, Fairmont Press, vol. 387, no 10016, , p. 86 (ISBN 978-0-88173-253-5, PMID 26231463, DOI 10.1016/s0140-6736(15)60078-2)
- (4.99 MB)
- (4.99 MB)
- Wet Cooling Tower Guidance For Particulate Matter, Environment Canada, Retrieved on 2013-01-29
- SPX Cooling Technologies MARLEY, Cooling Tower Fundamentals Page 73 (75 of 119) Column 2, Last Paragraph
- « SPX Cooling Technologies: Operating Cooling Towers in Freezing Weather » [archive du ] (1.45 MB)
- National Fire Protection Association (NFPA). NFPA 214, Standard on Water-Cooling Towers.
- NFPA 214, Standard on Water-Cooling Towers. Section A1.1
- National Fire Protection Association (NFPA). NFPA 214, Standard on Water-Cooling Towers.
- NFPA 214, Standard on Water-Cooling Towers. Section A1.1
- The use of water modulating by-pass systems is not recommended during freezing weather. In such situations, the control flexibility of variable speed motors, two-speed motors, and/or two-speed motors multi-cell towers should be considered a requirement.[1]
- Do not operate the tower unattended. Remote sensors and alarms may be installed to monitor tower conditions.
- Do not operate the tower without a heat load. Basin heaters may be used to keep the water in the tower pan at an above-freezing temperature. Heat trace ("heating tape") is a resistive heating element that is installed along water pipes to prevent freezing in cold climates.
- Maintain design water flow rate over the tower fill.
- Manipulate or reduce airflow to maintain water temperature above freezing point.[2]
Fire hazard modifier
Structural stability modifier
Being very large structures, cooling towers are susceptible to wind damage, and several spectacular failures have occurred in the past. At Ferrybridge power station on 1 November 1965, the station was the site of a major structural failure, when three of the cooling towers collapsed owing to vibrations in 85 Unité « mi/h » inconnue du modèle {{Conversion}}. ( Unité « km/h » inconnue du modèle {{Conversion}}.) winds.[réf. nécessaire] Although the structures had been built to withstand higher wind speeds, the shape of the cooling towers caused westerly winds to be funnelled into the towers themselves, creating a vortex. Three out of the original eight cooling towers were destroyed, and the remaining five were severely damaged. The towers were later rebuilt and all eight cooling towers were strengthened to tolerate adverse weather conditions. Building codes were changed to include improved structural support, and wind tunnel tests were introduced to check tower structures and configuration.[réf. nécessaire]
See also modifier
References modifier
External links modifier
- What is a cooling tower? – Cooling Technology Institute
- "Cooling Towers" – includes diagrams – Virtual Nuclear Tourist
- Wet cooling tower guidance for particulate matter, Environment Canada.
- Striking pictures of Europe’s abandoned cooling towers by Reginald Van de Velde, Lonely Planet, 15 February 2017 (see also excerpt from radio interview, World Update, BBC, 21 November 2016)
[[Catégorie:Technique de construction]] [[Catégorie:Chauffage, ventilation et climatisation]]